What are Working Principles and Characteristics of OTDRs?
The accuracy and utility of OTDR testing would not be possible without the science that preceded it.
It is a fiber optic instrument used to characterize, troubleshoot and maintain optical telecommunication networks. OTDR testing is performed by transmitting and analyzing pulsed laser light traveling through an optical fiber. The measurement is said to be unidirectional as the light is insert at extremity of a fiber optic cable link.
Using information obtained from the resultant light signature reflected or scattered back to the point of origin, the OTDR acts as an optical radar system, providing the user with detailed information on the location and overall condition of splices, connections, defects and other features of interest.
Find the right OTDR for you by using our OTDR Selector Tool.
The accuracy and utility of OTDR testing would not be possible without the science that preceded it. Understanding the physics behind the instrument provides invaluable insight into the working principles of OTDR.
When Albert Einstein theorized that electrons could be stimulated to emit a particular waveform, the seed of possibility that would eventually lead to the first operational laser in 1960 was born. While the applications envisioned at that time probably did not include worldwide telecommunications using fiber optics, this technology has now become synonymous with twenty-first century connectivity.
Over the years, many breakthrough discoveries have been leveraged in the development of OTDR testers.
OTDR Symbol Meanings
An OTDR contains a laser diode source, a photodiode detector and a highly accurate timing circuit (or time base). The laser emits a pulse of light at a specific wavelength, this pulse of light travels along the fiber being tested, as the pulse moves down the fiber portions of the transmitted light are reflected/refracted or scattered back down the fiber to the photo detector in the OTDR. The intensity of this returning light and the time taken for it to arrive back at the detector tells us the loss value (insertion and reflection), type and location of an event in the fiber link.
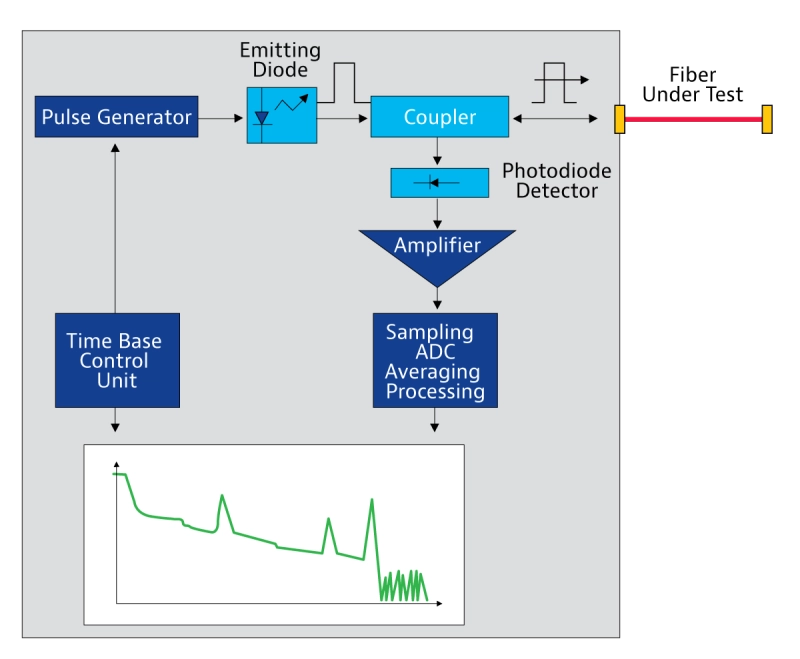
Light is returned to the photo detector through a number of mechanisms:
Rayleigh Scattering and Backscattering
Physicists of the previous century were still consumed with such fundamental questions as, “Why is the sky blue?” The answer to this question, as discovered by Lord Rayleigh in 1904, is what is now known as Rayleigh scattering. When light photons scatter off molecules in the air, the resulting light waves visible on the Earth are predominantly at the blue end of the spectrum because blue light is scattered more efficiently than red.
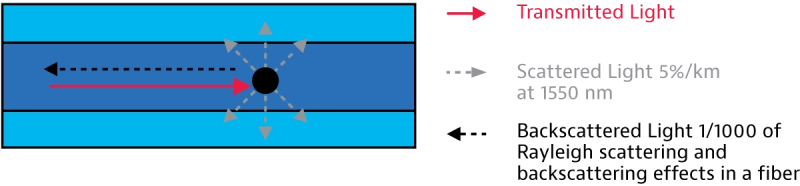
The predictable nature of Rayleigh scattering has been leveraged as a fundamental working principle in OTDR technology. The volume of source light energy backscattered to the detector provides a reliable indication of attenuation and signal (or optical) loss in the optical fiber link.
Fresnel Reflection
The properties of light reflection, characterized by optical physicist Augustin-Jean Fresnel, predated the discoveries of Rayleigh, but were equally important to the development of OTDR working principles.
Fresnel discovered the reflection coefficient which is a ratio of the reflected light wave amplitude relative to the original source wave. He found that the reflection coefficient could be predicted for the interface of two materials based on the respective refractive indices of these components.
Fresnel reflection occurs when light reflects off a boundary of two optically transmissive materials, each having a different refractive index. This boundary can occur at a joint (connector or mechanical splice), at a non-terminated fiber end, or at a break.
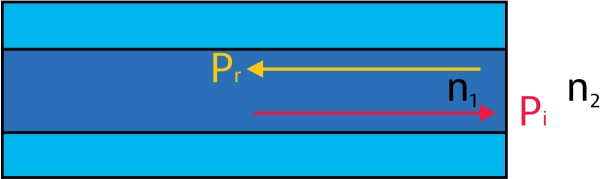
Since many events of interest in an optical fiber link, such as splices, breaks, connections and terminations, all represent specific material intersections such as glass and air, the Fresnel reflection equations can be used to determine the type, location and intensity of these events.
Absorption
Another physical property that is integral to fiber optic performance is the absorption of the fiber. As the name implies, a small percentage of the original light intensity is absorbed by internal impurities over the length of the fiber core. The greater the purity of the fiber, the less absorption will occur, meaning a higher quality material will result in less signal (or optical) loss.
Since the elements that induce absorption are inherently non-reflective, they would not be detected through Fresnel reflection measurements. Instead, the effects of absorption are captured through the backscatter effect, as the light returning to the source is absorbed proportionally to the incident light.
The inherent value of OTDR testing comes from diagnosing the condition of a fiber optic cable that would otherwise be impossible to see. This is essential when the link contains multiple splices and connections that can be subject to failure.
The optical return loss (ORL) and reflectance can be used to diagnose conditions where more loss than expected is occurring at a specific location in the fiber run. The total fiber attenuation can also be assessed, since the amount of backscatter provides an indication of this value.
These same principles are used to calculate distance measurements that are invaluable when repair, troubleshooting or maintenance needs arise. The end of the fiber link or a fiber break will be detectable through Fresnel reflection, since a break or unterminated fiber end is also a change in material media (glass to air). In addition to the overall length of the fiber, the distance to faults, splices and connections can be determined with a graphical presentation of the findings accompanying the analysis.
OTDR Types
As the functional utility of OTDR testing increases along with the demand for enhanced testing speed, accuracy, report generation and storage capabilities, the variation in product offerings continues to diversify. The two predominant categories are bench-top and hand-held. A bench-top OTDR is essentially a feature-rich instrument with a direct AC power source, whereas a hand-held or compact OTDR is typically a lightweight, battery-powered device intended for use in the field.
Beyond this basic division, the features and options available for an OTDR should be carefully considered based on the intended use. One important consideration is the type of fiber you will be testing - multimode, single-mode, or both. Another variable is the length of fiber you will be testing. Products designed for long haul applications typically have higher dynamic range capabilities that would not be required for testing shorter fiber optic links, such as FTTA.
Usability features also vary by product, which is yet another reason the intended application for the OTDR should be the most important factor in product selection (Import factors for choosing an OTDR). For example, a light weight product might not be necessary for a stationary test, but if the testing is going to be performed by technicians climbing cell towers or working in an otherwise active setting, weight, as well as features like battery life and ruggedization of the product enclosure become more important.
With the wide variety of applications for OTDR testing, setting parameters accurately for the task at hand will ensure accurate measurements. Using an auto-test function may be sufficient for some tests, but manual setting of parameters is still advisable given the variation in length, type, and complexity of optical fiber runs. Once the correct parameters for testing a given fiber run have been established, these OTDR testing configurations can be recalled from an instruments memory the next time the same or similar run is evaluated.
Pulse Width
Setting the adjustable pulse width determines the duration of the pulse being emitted into the fiber link. A shorter pulse width is usually selected for shorter cable lengths, since this will maximize resolution, while minimizing energy output. Short pulse widths are especially useful for evaluating segments of cable that are closer to the OTDR. Since these shorter pulse widths will also produce shorter dead zones, you will have a greater ability to detect events close to a connection or splice. Longer pulse width settings may be called for when testing a longer cable run, since more optical energy is required to produce sufficient backscatter at great distances from the OTDR.
Dead Zones
When the OTDR detector becomes saturated by a highly reflective interface in the fiber link, the recovery period for the OTDR translates to a distance from the event, known as a dead zone, which is essentially a portion of the cable for which no data will be available. Air gaps, bad splices, flat fiber end faces (connectors or the fiber end) and other incidences producing high Fresnel reflection are the usual causes of dead zones.
Distance Range
The distance range setting on an OTDR controls the display range for the amount of cable to be presented on the screen. It also defines the rate of pulse emission, since each pulse must be returned to the detector before the next pulse is sent out.
Setting this parameter appropriately requires accurate documentation of the optical fiber link. If the OTDR has preset distance range settings, you should choose the shortest setting that is still longer than the maximum fiber length. For example, if the instrument has settings of 10, 100, 200 and 500 kilometers, and your actual fiber link is 150 kilometers, you would select the 200 kilometer setting.
Averaging Time
In general, more accurate measurements are usually produced by averaging multiple repetitions of the same test. This same principle holds true with OTDR measurements. Longer averaging times, translating to more repetitions of the same test, will produce a measurement with an improved signal-to-noise ratio, but take longer to capture. For conditions where accuracy and noise are less critical, a “real-time measurement”, with no averaging function, could be sufficient. However, for circumstances where distance and loss data must be as precise as possible, longer averaging times might be justified.
Looking for more details about OTDRs?
Complete one of the following forms to get going: